Last of the most 'beautifully interesting' birds (part III)
Anyway, let's get on with it.
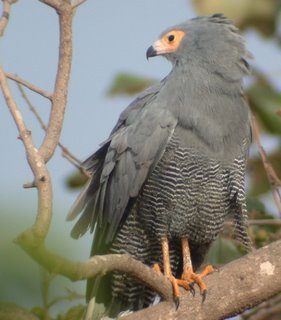
* I’m only discussing P. typus here, but there is another extant species in the genus (Madagascan P. radiatus).
Sometimes called the Gymnogene, Polyboroides is a gracile, naked-faced raptor with grey and black plumage, but best known for the so-called double-jointedness present in its intertarsal joints. The foot can actually bend both anteriorly and posteriorly (that is, the foot can be hyperextended as well as flexed), which needless to say is (almost) unique (read on). This is easy to see in dead or anaesthetized specimens, but it’s reportedly hard to observe in action in living birds. Cooper (1980) wrote that, after five months of observing captive specimens, the notebook was still being filled with such comments as ‘I can’t really fathom what is the ‘double-jointedness’ they refer to in the books’.
However, the behaviour has now been well documented and filmed, and the birds use this incredible flexibility to extricate lizards, insects and other prey from fissures in rocks, and nestling birds from their nests. You might presume, as I did, that Polyboroides has a unique sort of intertarsal joint, perhaps with the trochlear surfaces of the distal tibiotarsus wrapping onto the posterior surface of the bone as well as the anterior surface. But according to Cooper (1980) ‘in anatomical structure there is no significant difference between [the intertarsal joints of Polyboroides] and the corresponding joints of Kestrel, Tawny eagle or Black kite’ (p. 98). Err, gosh. I haven’t yet checked to see if there are any more recent studies of harrier-hawk ankles (let me know if you know better), but I find that pretty amazing. As I like to say sometimes 'Anatomy is not destiny' (not my quote, I stole it from a paper on armadillos).
Polyboroides is ‘beautifully interesting’ for two other reasons. Firstly, it’s amazingly similar to a South American raptor, the Crane hawk Geranospiza caerulescens. Geranospiza looks pretty much the same as Polyboroides, occupies the same ecological niche, behaves in the same manner, and even has the same bizarrely mobile intertarsal joint. Understandably, the two genera have often been regarded as each other’s closest relatives (e.g. Brown 1997). But…. they’re also different in many subtle anatomical details (Burton 1978), and consequently there’s been a long-standing debate as to whether they’re related or not. While unfortunately few phylogenetic studies include both species together, a comprehensive recent DNA-based phylogeny found the two to be well apart, with Polyboroides down with Old World vultures and honey buzzards, while Geranospiza was in the buteonine clade that also includes Buteo (of course) and Leucopternus (Lerner et al. 2005). This study is pretty compelling and the phylogeny near-conclusive. Polyboroides and Geranospiza thus represent striking, amazing instances of convergent evolution, one of the best examples of this among birds.
Incidentally, there's a second raptor that also evolved striking convergence with Polyboroides, but it's a fossil form and I'll have to talk about it another time.
Secondly, Polyboroides is interesting for being one of the most basal members of Accipitridae (the hawk-eagle-Old World vulture family). As hinted at in the previous paragraph, morphological and DNA-based phylogenies of Accipitridae tend to agree that one of the most basal clades in the group is that which includes Old World vultures and honey buzzards. Recent studies agree that Polyboroides is in this clade, and within it one of the most basal members (Holdaway 1994, Lerner et al. 2005). That makes it one of the most basal members of the whole of the accipitrid radiation. So you have there a raptor that (1) does something anatomically freaky, (2) does that anatomically freaky thing employing a mechanism that no-one yet properly understands, (3) exhibits uncanny, striking convergence with an unrelated raptor on another continent, and (4) is one of the most archaic, phylogenetically basal members of its group.
9. Kakapo Strigops habroptilis
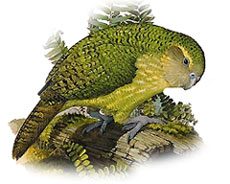
‘… [it] clambers up and down trees because it cannot fly … it purrs like a cat and smell like a posy of fragrant flowers … it allows itself to be picked up and handled without demur or apparent concern’ (Vietmeyer 1992, p. 69).
Sometimes called the Owl parrot, the Kakapo was first described by John Gray in 1845. Everything about kakapos is extraordinary. Remember that it’s a parrot while you read the following. A large nocturnal (!), cryptically-coloured (!!) terrestrial (!!!) bird, endemic to New Zealand, the kakapo is a specialized foliage-eater that seems to live on a metabolic knife-edge, rather like the Giant panda. Successful breeding is limited only to those years when mass fruitings of podocarps occur. This worked fine in a New Zealand where there were lots of podocarps and lots of kakapos (and indeed fossils show that kakapos were formerly abundant), but environmental destruction caused by humans meant that kakapos were forced into suboptimal areas where life was even harder than before. Combined with this was devastating predation from domestic cats, stoats and rats. Consequently, as is well known, kakapos have become extinct on the mainland and only survive on managed offshore islands where introduced rats and other predators have been eliminated (Vietmeyer 1992, Clout 2001). In 2001 there were 62 individuals. Worldwide.
Apparently in order to bulk-process the poor-quality vegetation it eats, the Kakapo has evolved voluminous guts, and accordingly a larger overall body size. It has hence become a giant among parrots (with big males reaching 3.6 kg), and is the largest extant species. Conventionally stated to be flightless, it is in fact capable of gliding, so this is not strictly true. Certainly it mostly walks places however, and individuals create well-worn trails in the mountainous forest where they live. Distinctively ‘chewed’, compressed fragments of vegetation hang from the plants adjacent to these trails, and kakapos leave both compact cylindrical droppings and white traces of uric acid on the trails. The uric acid streaks apparently ‘have a herb-like smell when fresh’ (Juniper & Parr 1998, p. 372).
Uniquely among parrots, kakapos are lek breeders, with males booming out loud calls from suitable topographical hollows, and these calls can be heard from about 1 km away. Cryptic plumage and nocturnal habits don’t make much sense in an environment devoid of predators, so the fact that kakapos possess these traits suggests that there were once predators able to kill them. Now we know that New Zealand did possess such predators: endemic giant eagles and large harriers (see New Zealand eagle blog).
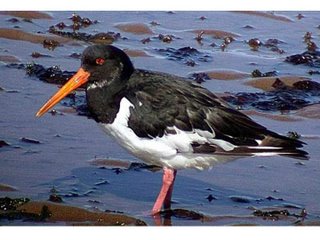
10. Eurasian oystercatcher Haematopus ostralegus
Finally, one of my most favourite birds is the extraordinary, charismatic, beautifully interesting oystercatcher. One of ten extant haematopodid species, it sports pied plumage, pinkish legs, and has the heaviest bill of any extant wader. One of the most interesting things about oystercatchers is the fact that they exhibit resource polymorphism, with some populations exhibiting multiple different forms (Skúlason & Smith 1995). ‘Stabbers’ feed by jabbing their laterally compressed bill tips in between the valves of a mussel’s shell, while ‘hammerers’ crack open mussel shells by pounding on them. Some hammerers only break in to the shell on its dorsal side, while others only break in to the ventral side. Others attack only the left side valve, and others only the right valve. Others are worm specialists with pointed tweezer-like bill tips.
First discovered by M. Norton-Griffiths during the 1960s (and extensively studied by a great many ornithologists since then), resource polymorphism among oystercatchers was initially thought to be learnt by the birds from their parents (and not genetically determined). It now seems that things are far more flexible, with individuals switching from one behaviour to the other over the years. It’s been said that juveniles can’t really learn how to handle prey from their parents given that many of them are reared inland and are abandoned by their parents before they ever get to the coast (Sutherland 1987). However, some oystercatcher adults spend up to a year teaching their young how to exploit prey: in fact a photo in Attenborough’s The Life of Birds shows an adult opening a shell while a juvenile, at its side, watches with apparent interest.
It seems that it’s the behavioural flexibility that controls bill shape, rather than the other way round, and another remarkable thing about oystercatchers is how specialized their bills are for coping with wear. Uniquely among waders, the bill grows at a jaw-dropping 0.4 mm per day (that’s three times faster than the growth rate of human fingernails). This rapid growth means that the bill can change shape very rapidly if the feeding style is changed, and captive individuals that were forced to switch from bivalve-feeding to a diet of lugworms changed from having chisel-shaped bills to tweezer-like bills within 10 days. A-maz-ing.
Given that oystercatchers are fairly large and powerful for waders, and able to smash open bivalve shells, it follows that they are formidable and potentially dangerous to other birds. Certainly males will chase off raptors when defending nesting females. I recall reading accounts of them caving in the heads of other waders during territorial disputes, but unfortunately I can’t remember where (a common problem, despite my well organized library). Most aggressive interactions recorded between oystercatchers, and between oystercatchers and other waders, involve piracy, and in fact some birds obtain most of their food this way, ‘attacking other birds at an average of five minute intervals during low tide’ (Hammond & Pearson 1994, p. 61). As much as 60% of the food of some individuals is obtained by piracy. Finally, oystercatchers are incredibly long-lived, with the record-holder dying at age 35! Now, come on, that is a truly extraordinary bird.
Tomorrow (June 1st) is viva day. Stay tuned for the news.
PS - for the latest news on Tetrapod Zoology do go here.
Refs - -
Brown, L. H. 1997. Birds of Prey. Chancellor Press, London.
Burton, P. J. K. 1978. The intertarsal joint of the harrier-hawks Polyboroides spp. and the Crane hawk Geranospiza caerulescens. Ibis 120, 171-177.
Clout, M. 2001. Where protection is not enough: active conservation in New Zealand. Trends in Ecology & Evolution 16, 415-416.
Cooper, J. E. 1980. Additional observations on the intertarsal joint of the African harrier-hawk Polyboroides typus. Ibis 122, 94-98.
Hammond, N. & Pearson, B. 1994. Waders. Hamlyn, London.
Holdaway, R. N. 1994. An exploratory phylogenetic analysis of the genera of the Accipitridae, with notes on the biogeography of the family. In Meyburg, B.-U. & Chancellor, R. D. (eds) Raptor Conservation Today. WWGBP/The Pica Press, pp. 601-649.
Juniper, T. & Parr, M. 1998. Parrots. Pica Press, Mountfield.
Lerner, H. R. L. & Mindell, D. P. 2005. Phylogeny of eagles, Old World vultures, and other Accipitridae based on nuclear and mitochondrial DNA. Molecular Phylogenetics and Evolution 37, 327-346.
Skúlason, S. & Smith, T. B. 1995. Resource polymorphisms in vertebrates. Trends in Ecology and Evolution 10, 366-370.
Sutherland, W. J. 1987. Why do animals specialize? Nature 325, 483-484.
- . 2002. Science, sex and the kakapo. Nature 419, 265-266.
Vietmeyer, N. D. 1992. The salvation islands. In Calhoun, D. (ed) 1993 Yearbook of Science and the Future. Encyclopaedia Brittanica Inc (Chicago), pp. 60-75.